Electric Field is the region around a charge in which another charge experiences an attractive or repulsive force. Electric Field is an important concept in the study of electrostatics which is the branch of physics. Electric Field despite its invisible nature, powers our homes with electricity, transportation system, as well as advancements in communication. Other than these roles, the electric field is also the driving force behind electric motors, propelling vehicles and machinery with remarkable efficiency. In this article, we will learn about Electric Field in detail, which helps us perform many daily activities with ease.
What is Electric Field?
The electric field exists everywhere in space and can be studied by introducing another charge into it. When an object carries an electric charge, it generates an electric field that affects the surrounding area in a way such that, other charged particles around it experience a force either of attraction or repulsion. Also, the distance between these charge particles also affects the magnitude of force experienced by these particles. Let’s define the electric field first in the following heading.
Electric Field Definition
An electric charge creates an electric field, which is a region of space around an electrically charged particle or object where the charge feels forced.
If the charges are far enough apart, the electric field can be approximated as 0 for practical purposes. The electric field is a vector quantity represented by arrows pointing toward or away from charges.
Note: Electric Field Lines must point radially outward, away from a positive charge, or radially inward, toward a negative charge.
What is an Electric Charge?
Electric charge is a fundamental property of matter that causes it to experience a force when placed in an electromagnetic field. There are two types of electric charges: positive and negative. Like charges repel each other, while opposite charges attract.
Electric Charge Definition
Electric charge is a basic attribute of subatomic particles that leads to forces acting on them within electric and magnetic fields. These fields impact charged particles, producing measurable effects.
Electric Field Strength
The electric field strength at a point in space is defined as the force experienced by a unit positive charge placed at that point. In other words, the magnitude of the strength of the electric field is called Electric Field Strength and it can be calculated using the formula:
E = F/q
Where,
- E is the electric field’s strength,
- F is the electric force, and
- q is the test charge.
Types of Electric Charge
Electric charge is classified into two primary types: positive and negative. Positive charge is associated with protons, subatomic particles found in an atom’s nucleus, and is indicated by the “+” symbol. Conversely, negative charge is linked to electrons, which orbit around the nucleus, and is represented by the “-” symbol.
Understanding the distinction between positive and negative charges is crucial for explaining how electrically charged objects behave. Opposite charges attract, meaning positive and negative charges pull towards each other, while similar charges, like positive with positive or negative with negative, repel each other. This fundamental behavior underlies many concepts in electromagnetism and is essential for comprehending the interactions between charged particles.
An object with a negative charge has more electrons than protons, while an object with a positive charge has more protons than electrons.
When an object has an equal number of positive and negative charges, these charges neutralize each other, rendering the object electrically neutral.
Recognizing the role of electric charge and the dynamics of positive and negative charges is vital for understanding the basic principles of electricity and magnetism.
In the study of electric charges, “attraction” and “repulsion” describe the interactions between charges.
Check: Electric Charge Formula
Unit of Electric Field Strength
The unit of electric field strength is volts per meter (V/m) which represents the amount of electric potential difference (voltage) per unit length. Some other units of Electric Field Strength include Newtons per coulomb (N/C) [derived from the above-mentioned formula i.e., E = F/q] and Statvolts per centimeter (statV/cm) [in cgs system], where 1 statvolt/cm is equal to 3.3356 V/m.
Check: Unit of Electric Charge
Coulomb’s Law
Coulomb’s Law describes the forces exerted by point charges on each other. In physics, “Point Charge” refers to the fact that linearly charged objects have a small size in contrast to the distance between them. As a result, we treat them as point charges since calculating the attraction/repulsion force between them is straightforward.
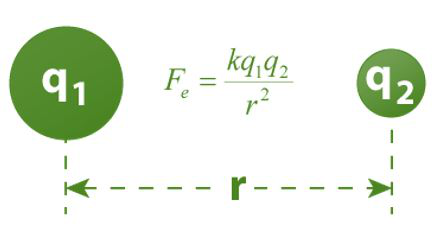
Pictorial Representation for Coulomb’s Law
The statement, in general, includes two charges, q1 and q2. The attraction/repulsion force between the charges is denoted by the letter ‘F,’ while the distance between them is denoted by the letter ‘r.’ Then Coulomb’s law is expressed mathematically as-
- F is proportional to the product of the magnitudes of the charges in contact, i.e. F ∝ q1q2.
- F is inversely proportional to the square of the distance between the two charges in contact, i.e. F ∝ 1/ r2.
Let’s put the two together as follows:
F ∝ q1q2 / r2
Now, if we remove proportionality a constant k is introduced;
F = k q1q2/ r2
Where
- k=1/4πεo is the proportionality constant, [equals to 9 × 109 Nm2/ C2.]
- εo represents the permittivity of free space i.e., vacuum.
According to Coulomb, like charges repel one other whereas unlike charges attract each other. This indicates that charges of the same sign repel one another, whereas charges of opposite signs attract one another.
Representation of Electric Fields
There are two ways in which we can represent the Electric Fields
- Electric Field Lines
- Electric Field Vectors
Let’s discuss these representations in detail as follows:
Electric Field Lines
Electric Field Lines are the imaginary line representing the electric field. These Field Lines have the following characteristics:
- Electric Field Lines are continuous curves.
- They begin with a positively charged body and end with a negatively charged one.
- The direction of electric field intensity at any point is determined by being tangent to the electric field line.
- There are no two electric field lines that cross each other.
- Electric field lines are always parallel to the conductor’s surface.
Learn more about Electric Field Lines.
**Image of Electric Field Lines and Electric Field Vectors
Electric Field Vectors
Similar to electric field lines, electric field vectors are another way in which we can represent the direction and magnitude of an electric field. At each given point, the electric field vector indicates the direction of acceleration of a positive test charge if placed at that point in the electric field. Electric field vectors are represented the same as vectors i.e., using arrows where the length of the arrow represents the magnitude of the electric field and the direction of the vector represents the direction of the electric field.
Some key characteristics of the electric field vectors are:
- The direction of the electric field vector is the same as the direction in which a positive test charge would move if placed at that point.
- The magnitude of the electric field vector is proportional to the strength of the electric field at that point.
- Electric field vectors point away from positive charges and towards negative charges.
Check: Electric Field Formula
Electric Field Due to Point Charges
The electric field due to a point charge is a vector field that describes the electric field strength and direction at different points in space surrounding the charge. The electric field vector at a given point represents the force experienced by a positive test charge placed at that point.
**Image of Electric Field Lines Due to Positive Point Charge and Negative Point Charge
The electric field (E) due to a point charge (Q) at a distance (r) from the charge can be calculated using Coulomb’s law, and the formula for that is given as follows:
E = k × (Q/r2)
Where
- E is the electric field strength,
- k=1/4πεo is the proportionality constant, [equals to 9 × 109 Nm2/ C2.],
- Q is the magnitude of the point charge, and
- r is the distance from the charge to the point where the electric field is being calculated.
Note: The above formulas hold true for point charges, which are considered to have negligible size compared to the distance at which the electric field is being calculated.
Learn more about Electric Field Due to Point Charges.
Electric Field Due to Continuous Charge Distributions
The electric field due to a continuous charge distribution is calculated using integration techniques, as the charge is spread out over a continuous region rather than being concentrated at a single point. To find the required electric field due to the region of Continuous Charge Distributions at a point, we integrate the electric field contribution of infinitesimally small charge elements.
For a continuous charge distribution, the electric field (E) at a point can be calculated using the following integral:
E = ∫ (k × dq / r2)
Where:
- E is the electric field vector at the point,
- k=1/4πεo is the proportionality constant, [equals to 9 × 109 Nm2/ C2.],
- dq is an infinitesimal charge element within the continuous charge distribution, and
- r is the distance from the charge element to the point.
In this the charge distribution can be one-dimensional i.e., linear charge distributions (e.g., charged wires), two-dimensional i.e., surface charge distributions (e.g., charged plates), or three-dimensional i.e., volume charge distributions (e.g., charged spheres or cubes), depending on the geometry of the system.
Learn more about Continuous Charge Distribution.
Consider a line segment of length L with a uniform charge density λ. We want to determine the electric field at a point P located a distance r away from the line.
**Image of the uniformly Charged Line**
Let’s consider a small charge element of length dx located at a distance x from point P. The charge dq of this element is given by dq = λ dx.
The electric field dE at point P due to this small charge element can be calculated using Coulomb’s law:
dE = \frac{1}{4\pi\epsilon_0} \cdot \frac{dq}{r^2}
where εo is the permittivity of free space.
Since the charge element dq is distributed uniformly along the line, we can express dq in terms of x as:
dq = \lambda \cdot dx
Substituting this into the equation for dE, we get:
dE = \frac{1}{4\pi\epsilon_0} \cdot \frac{\lambda \cdot dx}{r^2}
To find the total electric field at point P, we integrate dE over the entire length of the line from x = -\frac{L}{2} to x = \frac{L}{2}:
[Tex]E = \int_{-\frac{L}{2}}^{\frac{L}{2}} \frac{1}{4\pi\epsilon_0} \cdot \frac{\lambda \cdot dx}{r^2}
[/Tex]
Integrating this expression will give you the electric field E at point P due to a uniformly charged line.
Note: The direction of the electric field will be radial, pointing away from the line if the line is positively charged and toward the line if the line is negatively charged.
Learn more about Electric Field Due to Infinite long Straight Wire.
Consider a uniformly charged ring radius R with zero thickness and total charge +Q. Let’s assume we want to calculate the electric field at a point P located on the axis of the ring at a distance x from the center of the ring, O.
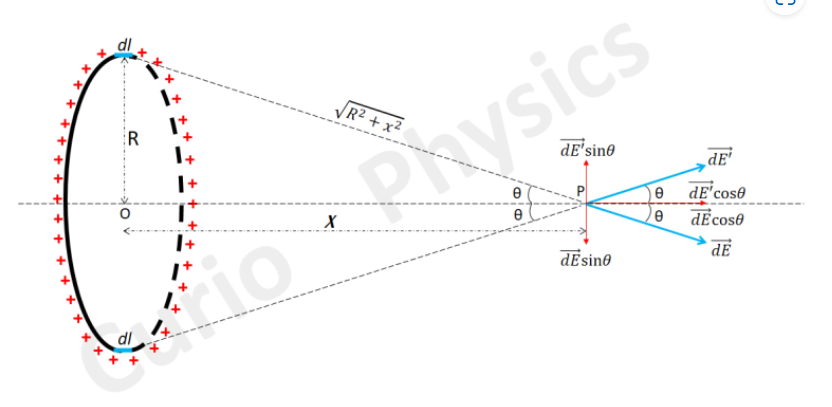
rough diagram
Let us consider a circular ring of wire with zero thickness and a radius R. +q is the charge on the ring, distributed uniformly over the ring’s circumference. We have to determine the electric field intensity at any point P on the axis of the loop, which is at a distance x from the center of the ring O.
Let us consider a small element of the ring of length dl.
and the charge on this element is dq, then
dq =qdl/2πR
Where q/2πR is the charge per unit length.
Electric field intensity at P due to charge element dl, Using the Electric Field Due to Continuous Charge Distributions, is,
dE = k dq/r2
Where,
- k is the electrostatics constant = 1/4πεo, and
- r is the distance between the charge element and Point P.
⇒ dE =k dq/(R2 + x2)1/2
To simplify, resolve electric field intensity dE into two rectangular components, dE sin θ (along the y-axis) and dE cos θ (along the x-axis) as shown in the diagram.
For the pair of diametrically opposite elements of the charged ring, perpendicular components of the electric field intensity will cancel each other. i.e.,
∫dE sinθ = 0.
Whereas components along the axis of the charged ring will be integrated. That is,
∫dE cosθ.
Hence, the resultant electric field intensity E at P is | E | = ∫dE cosθ
In \triangle OPA,
\cos \theta=\mathrm{OP} / \mathrm{CP}=\frac{x}{\sqrt{R^2+x^2}}
Therefore,
\begin{aligned}
& |\mathrm{E}|=\int \frac{k x d q}{\left(R^2+x^2\right) \sqrt{R^2+x^2}} \\
& |\mathrm{E}|=\frac{k q x}{\left(R^2+x^2\right)^{3 / 2}}
\end{aligned}
The direction of E is along the positive x-axis of the loop.
Special Cases
1. When point P lies at the center of the loop
x=0, therefore,
from |\mathrm{E}|=\frac{k q x}{\left(R^2+x^2\right)^{3 / 2}},
E=0
2. When x \gg p
|E|=\frac{k q x}{\left(R^2+x^2\right)^{3 / 2}}, since x \gg>R so neglect R^2, we get |\mathrm{E}|=\frac{K q x}{x^3}=\frac{K q}{x^3}
To find the electric field at point P due to the disk, let us consider an elemental ring of radius y and width dy if dq is the charge on this infinitesimal element, then
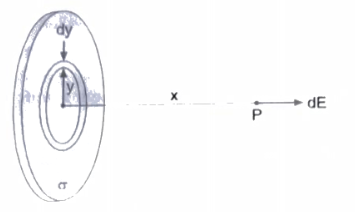
rough image
dq = \sigma dA
dq = \sigma (2 \pi y d y)
[Area of element is dA = 2 \pi y d y
and \sigma is the uniform current density of the disk.
Now we know that electric field strength due to a ring of radius R, charge Q, at a distance x from its center on its axis can be given as
E_{axis}=\frac{1}{4 \pi \epsilon_0} \frac{Qx}{\left(x^2+R^2\right)^{3 / 2}}
So, due to the infinitesimal elemental ring the electric field strength dE at point P is
\begin{aligned}
dE=\frac{1}{4 \pi \epsilon_0}\frac{(dq)x}{\left(x^2+y^2\right)^{3 / 2}} \\
\Rightarrow dE = \frac{1}{4 \pi \epsilon_0}\frac{ \sigma (2 \pi y d y)x}{\left(x^2+y^2\right)^{1 / 2}}
\end{aligned}}
Here, as we already chose the point P, thus x remains constant under the integral.
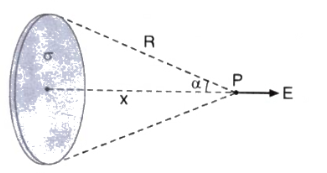
rough image
\begin{aligned}
& E=E_{\text {axis }}=\int_0^R d E \\
\Rightarrow & E=\int d E=\frac{1}{4 \pi \varepsilon_0} \int_0^R \frac{\sigma 2 \pi x y d y}{\left(x^2+y^2\right)^{3 / 2}} \\
\Rightarrow & E=\frac{\sigma \pi x}{4 \pi \varepsilon_0} \int_0^R \frac{2 y d y}{\left(x^2+y^2\right)^{3 / 2}} \\
\Rightarrow & E=\frac{\sigma x}{4 \pi \varepsilon_0}\left[-\left.\frac{2}{\sqrt{x^2+y^2}}\right|_{y=0} ^{y=R}\right] \\
\Rightarrow & E=\frac{\sigma}{2 \varepsilon_0}\left[1-\frac{x}{\sqrt{x^2+R^2}}\right]
\end{aligned}
To calculate the electric field due to a uniformly charged sphere at a point outside the sphere, we can use the principles of symmetry and integration.
Consider a uniformly charged sphere with radius R and total charge Q. We want to find the electric field at a point P located outside the sphere, at a distance r from the centre of the sphere.
To find the electric field at point P, we can divide the sphere into infinitesimally small charge elements. Each charge element will contribute to the electric field at point P.
The electric field due to an infinitesimal charge element dQ is given by Coulomb’s law:
dE = (k × dQ) / r^2
where k is the electrostatic constant (k ≈ 8.99 × 10^9 N m^2/C^2), dQ is the charge element, and r is the distance from the charge element to point P.
Since the sphere has spherical symmetry, we can consider a spherical shell on the sphere with radius r and thickness dr, representing an infinitesimal charge element. The charge element dQ on this shell is related to the total charge Q and the surface area of the sphere.
dQ = (Q / (4πR^2)) × (4πr^2 × dr)
Substituting dQ into the equation for dE, we get:
dE = (k × Q × dr) / (4πε₀ × r^2)
To find the total electric field at point P, we integrate the contributions from all the infinitesimal charge elements on the sphere. The integral is taken over the entire surface of the sphere.
E = ∫ dE = ∫ (k × Q × dr) / (4πε₀ × r^2)
The limits of integration are from the radius of the sphere, R, to the distance from the center of the sphere to point P, r.
Integrating this expression gives us the total electric field due to the uniformly charged sphere at point P outside the sphere.
If the point P is inside the sphere (r < R), the electric field due to the charged sphere is zero at the center of the sphere and increases linearly with distance from the center until it reaches the surface of the sphere. Inside the sphere, the electric field is given by:
E = (k × Q × r) / (4πε₀ × R^3)
For points inside the sphere, the electric field is directly proportional to the distance from the center and depends on the total charge and radius of the sphere.
It’s important to note that the above calculations assume a nonconducting sphere. In the case of a conducting sphere, the charge distribution and resulting electric field may differ due to charge redistribution on the surface of the conductor.
Methods of Charging
The act of charging involves either supplying electric charge to an object or enabling it to lose electric charge. There are three primary ways an initially uncharged object can acquire a charge:
- Charging by friction
- Charging by conduction
- Charging by induction
Charging by Friction:
This method occurs when two objects are rubbed together, causing electrons to transfer from one object to the other. The object that loses electrons becomes positively charged, while the object gaining electrons becomes negatively charged. This effect is also known as electrification by friction.
Charging by Conduction:
In this approach, an uncharged object is placed near a charged object, leading to a transfer of electrons to balance out an uneven number of protons and electrons. This contact-based charge transfer is known as charging by conduction.
Charging by Induction:
Charging by induction happens when an uncharged object is brought near a charged object without direct contact. The presence of the charged object causes a redistribution of charges in the uncharged object, enabling it to acquire a charge.
Understanding these methods enriches our knowledge of how objects are charged through different interactions such as rubbing, contact, or proximity, and provides insights into the dynamics and behavior of electric charges under various conditions.
Properties of Electric Charge
Electric charge exhibits several key properties that enhance our understanding of its behavior.
Additivity of Electric Charge:
Charges, when combined, sum algebraically. For instance, merging a positive charge of +3 units with a negative charge of -2 units results in a net charge of +1 unit.
Conservation of Electric Charge:
Within an isolated system, the electric charge remains conserved. This indicates that the overall electric charge of the system is constant over time, maintaining the same algebraic sum of all present charges.
Quantization of Electric Charge:
Electric charge is quantized, meaning it appears in fixed, indivisible units known as elementary charges. The smallest recognizable charge unit is the electron’s charge, approximately -1.6 x 10^-19 coulombs. This property suggests that charges cannot be subdivided further.
These principles aid in grasping the dynamics of electric charges and their significance in various scientific contexts.
Check: Basic Properties of Electric Charge
Sample Problems on Electric Field
Problem 1: A charge of 2 C is placed in the center of an 8 cm3 cube. What is the magnitude of the electric flux traveling through one of the faces?
Solution:
Given,
- Cube’s volume = 8 cm3
- Side Length = 2 cm
Electric flux over a closed surface is contained charge within the closed surface divided by the medium’s permittivity, according to Gauss’s theorem.
If the medium within the cube is air or vacuum, the total electric flux over the closed surface is
Φ = Q / εo
Φ = 2 / (8.854 10-12)
Φ = 2.259 1011 N C-1 m2
Electric flux passing through one face is ( 2.259 × 1011 ) / 6 = 3.765 × 1010 N C-1 m2
Problem 2: A force of 8 N is experienced when two point charges separated by 1 m have equal charges. What force will they feel if they are both submerged in water at the same time? (Assuming K water = 80)
Solution:
Force acting between two-point charges
Fair = q1q2/4πεor2
Fwater = q1q2/4πkεor2
Therefore,
Fair/Fwater = K
8/Fwater = 80
Fwater = 8/80
Fwater = 1/10N
Problem 3: Consider a system of two charges of magnitude 3 × 10-7 C and 4 × 10-7 C which are acted upon by a force of 0.1 N. What is the distance between the two charges?
Solution:
Given,
- First charge q1 = 3 × 10-7 C
- Second charge q2 = 4 × 10-7 C
- Force F = 0.1 N
The formula to calculate the electrostatic force between the charges is:
F = k q1q2 /r2
Substitute the given values in the above expression as
0.1 N = (9 × 109 Nm2/ C2)(3 × 10-7 C)(4 × 10-7 C) / (r)2
r = 0.103 m
Hence, the distance between the two charges, r is 0.103m.
Electric Field – FAQs
Define Electric Field.
An electric field is a physical field that surrounds electrically charged particles or objects in which any other charged charged particle experience an electrostatic force.
How is an Electric Field Represented?
Electric fields are represented using electric field lines or vectors, where field lines and vector shows thier magnitude and direction.
What is Electric Field Formula?
The formula for the electric field (E) due to a point charge (Q) at a distance (r) is given as:
E = k(Q/r2)
Where,
- E is the electric field strength,
- k=1/4πεo is the proportionality constant, [equals to 9 × 109 Nm2/ C2.],
- Q is the magnitude of the point charge, and
- r is the distance from the charge to the point where the electric field is being calculated.
What are the Units of the Electric Field?
The SI unit of electric field is newtons per coulomb (N/C). Another common unit is volts per meter (V/m). Electric field can also be expressed in terms of other units such as statvolts per centimeter (statV/cm) in the cgs (centimeter-gram-second) system.
Can Electric Field be Negative?
Yes, the electric field can be negative. The electric field is a vector quantity, which means it has both magnitude and direction. The sign of the electric field indicates the direction of the force that a positive test charge would experience if placed in that field.
Can an Electric Field Exist in a vacuum?
Yes, an electric field can exist in a vacuum. Electric fields are present in empty space even in the absence of matter. For example, electromagnetic waves such as light consist of oscillating electric and magnetic fields that propagate through a vacuum.
Are Electric Fields the Same as Magnetic Fields?
Electric fields and magnetic fields are two different phenomena as electric fields are produced by electric charges, while magnetic fields are created by moving charges or currents. However, changes in electric fields can generate magnetic fields, and vive versa is also possible.
Why Electric Field Inside a Conductor is Zero?
Due to electrostatic shielding effect, electric field inside a conductor is zero.
Why Electric Field is Perpendicular to Equipotential Surface?
An equipotential surface is a surface on which the electric potential at all the points remains the same i.e., there is no potential difference across the surface. Due to this electric field lines are always perpendicular to the equipotential surfaces because if there were any other components of the electric field which is non-perpendicular to the equipotential surface, it would cause a change in electric potential along that surface which is not possible due to the definition of equipotential surface.
Does Electric Field Exist in Empty Space?
Yes, electric fields can exist in empty space as there is only one thing needed for an electric field to exist i.e., electric charge and electric change can exist in empty space. However, in the absence of any charges, the electric field would be zero.
Share your thoughts in the comments
Please Login to comment...