Electric field is a fundamental concept in physics, defining the influence that electric charges exert on their surroundings. This field has both direction and magnitude. It guides the movement of charged entities, impacting everything from the spark of static electricity to the functionality of electronic devices Understanding electric fields will help you to understand how charge particles interact with each other and the surroundings and guide various natural and technological phenomena. In this article, we will learn in detail about electric field, its formula, calculation of electric field for ring, straight wire and continuous charge distribution.
What is an Electric Field?
Electric Field is a fundamental concept in physics, they are a physical field that surrounds an electrically charged particle. Charged particles exert attractive force when they have opposite charges and repulsive force when they have the same charges in this field. The electric field of a single charge describes its power to exert such forces on another charged object.
The electric field is a vector quantity which means it has both magnitude and direction.
Mathematically electric field(E) is described at a given point is defined as the force (F) experienced by a test charge (q) placed at that point, divided by the magnitude of the test charge:
E = F/q
Direction Of Electric Field
The direction of electric field is taken as the direction of the force which is exerted on the positive charge. The electric field is radially outwards from the positive charge and radially inwards to the negative point charge, as shown in the picture given below:
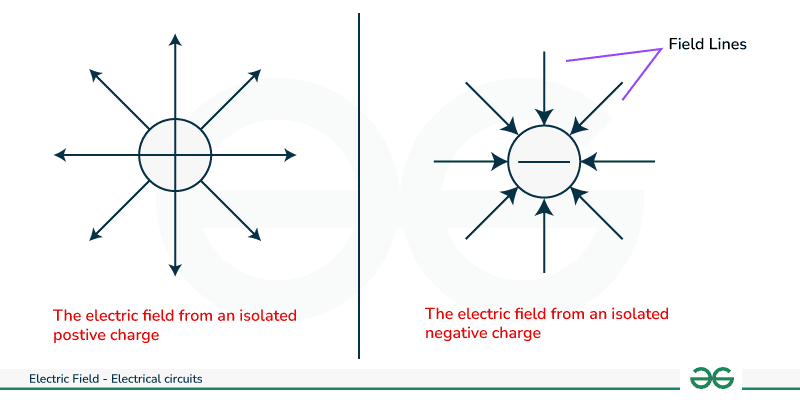
Electric Field Lines
Now, let us see some of the important terminologies related to electric field that will be discussed further in the article:
- Electric Charge: An electric charge is a property of matter that causes two objects to repel or attract. It can be either negative or positive.
- Point charge: When discussing a body considerably smaller than the distance being considered, we disregard its size and refer to it as a point charge.
- Coulomb’s Law: Coulomb’s law states that the force between two point charges is directly proportional to the magnitudes of the charges and inversely proportional to the distance between the two charges.
- Mathematically : F = (k|q1q2|)/r2 ,where q1=first point charge ,q2 = second point charge.
- k = 8.988 * 109 Nm2/C2 is Coulomb’s constant, r = the distance between two point charges.
- Gauss’s law : Gauss’s law states that the net flux of an electric field in a closed surface is directly proportional to the enclosed electric charge.( further discussed in detail in the article).
- Electric flux: Electric flux is defined as the total number of electric field lines passing through a specified area within a unit of time.
Electric Field Lines
Electric field lines are imaginary lines used to depict the direction and strength of an electric field surrounding charged objects. They were introduced by the physicist Michael Faraday in the 19th century as a conceptual tool for understanding electric fields.
Properties of Electric Field Lines
Electric field lines have different properties. Some of the properties are provided below:
- Field lines never intersect each other.
- They are perpendicular to the surface charge.
- The strength of an electric field increases when the field lines are closer together, which indicates a stronger force. Conversely, when field lines move farther apart, the field weakens.
- The quantity of field lines directly proportional to the magnitude of the charge.
- These lines generally originate from positive charges and ends at negative charges, depicting the direction of the electric field.
Electric Field Calculation
Various methods are followed to calculate electric field are as followed:
Calculate Electric Field Using Coulomb’s Law
According to Coulomb’s law, a force with electric charge q1 at position x1 exerts a force on a particle with charge q2 at position x0 of ,(equation-1)
[Tex] \vec F= \frac{1}{4\piε_0}\frac{Q.q}{r^2}\hat r[/Tex]
where
- [Tex]\hat r[/Tex] is a unit vector in the direction from of electrostatic force
- ε0 is the electric constant, known as absolute permittivity of free space .
We know that electric field is given as force per unit test charge. Let q is the test charge. Hence, electric field is given as
[Tex]\vec E = \frac {\vec F}{q} = \frac{1}{4\pi\varepsilon_0} \frac {Q}{r^2}\hat r[/Tex]
Calculate Electric Field Using Gauss’s Law
Gauss’s law states that the total flux linked with a closed surface is 1/ε0 times the charge enclosed by the closed surface. Hence, according to Gauss Law, electric field is given as follows:
[Tex] ∮ \overrightarrow{\rm E}.\overrightarrow{\rm ds}= \frac {q}{\varepsilon_0}[/Tex]
It is easy to calculate electric field using Gauss’s law as compare to Coulomb’s law. If we carefully observe, Gauss’s law is a replica of Coulomb’s law.
How to Find the Electric Field Using Gauss Law?
- Choose a Symmetrical Gaussian Surface: Select a closed surface where the electric field is constant in magnitude and direction. The symmetry can be spherical, cylindrical or planar depending upon the charge distribution.
- Determine the Enclosed Charge: Calculate the total charge enclosed in the chosen Gaussian surface.
- Calculate Electric Flux: Evaluate the flux of the electric field through the Gaussian surface
- Ultimately leads to finding the electric field using gauss law with the help of the formula given above.
Let’s say you have a spherically symmetric charge distribution with total charge Q uniformly distributed within a sphere of radius R. Our aim is to find electric field at a distance r from the center:
- Choose a Gaussian Surface: A spherical Gaussian surface with radius r > R is suitable for this case.
- Determine Enclosed Charge: The enclosed charge is q for r ≤ R and Q for r > R (as whole body is enclosed).
- Calculate Electric Flux: The electric flux through the spherical surface is given as E × A, where E is the magnitude of the electric field and A is area
- Apply Gauss’s Law: By Set up Gauss’s law equation:
[Tex] ∮\overrightarrow{\rm E}.\overrightarrow{\rm dA} = \frac {q_{enclosed}}{\epsilon_0}[/Tex]
From Gauss Law
4πr2.E = Q/ε0
From this equation, you can solve for the magnitude of the electric field
E at a distance r from the center is given as
[Tex]\vec E = \frac {1}{4\pi \epsilon_0} \frac {Q}{r^2}[/Tex]
This example illustrate how to use Gauss’s law for a spherically symmetric charge distribution. See, the approach and the shape of the Gaussian surface can vary based on the symmetry of the charge distribution provided in the question.
Electric Field For Continuous Charge Distribution
Let us talk about the different types of charge distribution
- Linear Charge Distribution
- Surface Charge Distribution
- Volume Charge Distribution
Linear Charge Distribution
Linear charge distribution: If charge is distributed linearly over a body.
λ = dq/dl
- λ = linear charge density
- dq = charge
- dl = line element
Consider an infinitesimally small element of charge (dq) along the line, the electric field contribution (dE) from this element can be calculated using Coulomb’s law: dE = k⋅dq/r2 where k is Coulomb’s constant.
Integrate dE over the entire length of the line charge to obtain the total electric field E = ∫k⋅dq/r2
Surface Charge Distribution
Surface charge distribution: If charge is distributed continuously over the surface of a body
σ = dq/ds
- σ = surface charge density
- dq = charge
- ds = surface element
Consider an infinitesimally small element of charge (dq) on the surface, the electric field contribution (dE) from this element can be calculated using Coulomb’s law: dE = k⋅dq/r2, where k is Coulomb’s constant.
Integrate dE over the entire surface to obtain the total electric field E = ∫k⋅dq/r2
Volume Charge Distribution
Volume charge distribution: If charge is distributed continuously over the volume of a body.
ρ = dq/dv
- ρ = volume charge density
- dq = charge
- dv = volume element
Consider an infinitesimally small volume element containing charge (dV) within the region, the electric field contribution (dE) from this volume element can be calculated using Coulomb’s law: dE = k⋅dq/r2, where dq = ρ⋅dV and k is Coulomb’s constant.
Integrate dE over the entire volume to obtain the total electric field E = ∫k⋅dq/r2
Applications of Gauss Law to Find Electric Field
Now, let us see some of the application of gauss law to find electric field:
Electric Field due to a Line Charge
Suppose a line charge having linear charge density λ is given in the form of a thin charged rod.
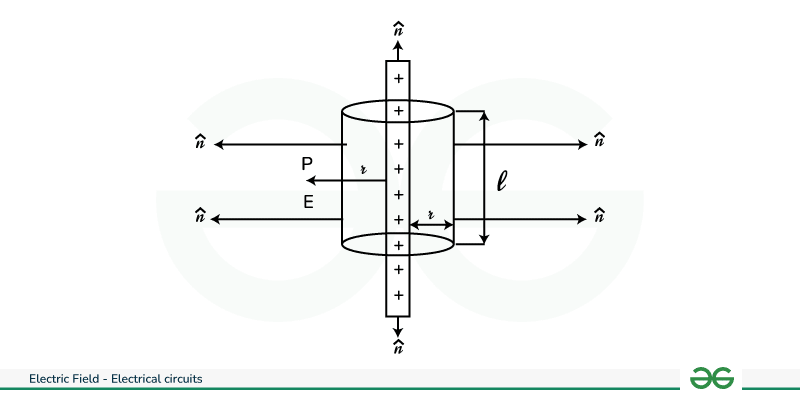
Diagram for line charge
To find the electric field intensity at point P along a wire, a cylindrical Gaussian surface is selected. This choice is made to apply Gauss’s law for finding the electric field, E, at point P.
The electric flux passing through the end surfaces of the cylindrical Gaussian surface is ,that is Φ1 = 0.
And , the electric flux passing through the curved surface of the cylindrical Gaussian surface is given as:
Surface area of curved part is given as: S = 2πrl
Total charged enclosed by gaussian surface is q = λ × l
Surface area and charge of gaussian surface (Equation-5)
The electric flux through the curved surface of the cylindrical Gaussian surface is given as:
[Tex]\phi = \overrightarrow{\rm E}.\overrightarrow{\rm dS}
[/Tex]
Φ = E. cos θ.S = E × 1 × 2πrl
Total electric flux is given as:
Φ = Φ1 + Φ2
Φ = 0 + E.cos θ.S
Φ = E × 2πrl
From Gauss’s law, we know that,
Φ = q/ε0 = λl/ε0
2πrl × E = λl/ε0
[Tex]E = \frac {1}{2\pi\epsilon_0} \frac {\lambda}{r}[/Tex]
Electric Field Due to Ring
Now, let us take a look into the electric field due to Ring
For, Electric Field Intensity at Any Point on the Axis of a Uniformly Charged Ring, let us consider a wire forming a circular ring with negligible thickness and a radius of R, carrying a uniform charge +q distributed evenly around its circumference. Our aim is to calculate the electric field intensity at any point P along the axis of the loop, positioned at a distance x from the ring’s center, marked as O.
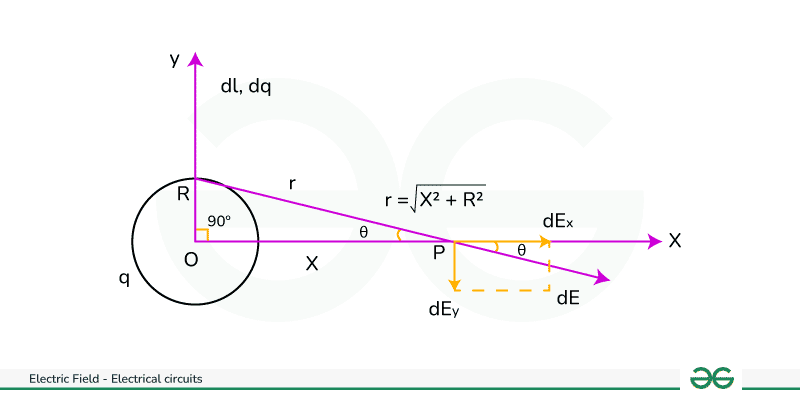
Diagram showing electric field due to charged ring
Let AB be the length of element dl.
The charge on the element AB is,
dq = (q × dl)/2πR
Electric field intensity at P due to charge element AB is,
|dE| = k dq/(CP)2
where, k = constant
|dE| = k dq/(R2 + x2)
Now, resolve the electric field intensity dE into two rectangular components, that is
dE sinθ along the y-axis and dE cosθ along the x-axis.
And for diametrically opposite elements of the charged ring, the perpendicular components of the electric field intensity will nullify each other, resulting in,
∫dE sinθ = 0.
Whereas components along the axis of the charged ring will undergo integration. That is, ∫dE cosθ.
Hence, the resultant electric field intensity E at P is | E | = ∫dE cosθ
In △OPC, cos θ = OP/CP = x/√(R2 + X2 ) and |dE| = k dq/(R2 + x2)
Therefore, |E| = ∫k × x × dq/(R2 + X2 )(√(R2 + X2 )
|E| = k × q × x/(R2 + X2 )3/2
The direction of E is along the positive x-axis of the loop.
Now, let us talk about the electric field due to a uniformly charged sphere.
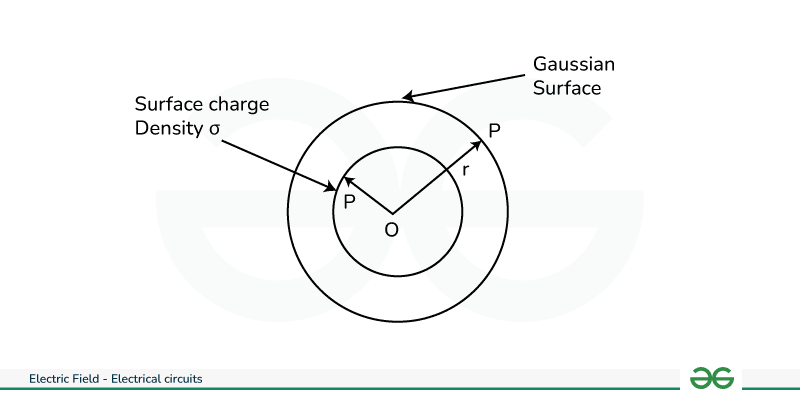
Charged sphere
Electric Field Outside the Shell
To find out the electric field intensity at a point P outside the spherical shell when OP = r.
The Gaussian surface was taken as a sphere having radius r, while the electric field intensity will remain the same at every point seen on the Gaussian surface.
Thus, Gauss theorem becomes,
∮ [Tex]\overrightarrow{\rm E}.\overrightarrow{\rm dS}
[/Tex]= ∮[Tex]\overrightarrow{\rm E}.\hat ndS = \frac {q}{\varepsilon_0}[/Tex]
or it can be also be given as,
E∮dS = q/ε0
E × (4πr2) = q/ε0
Therefore, the electric field become,
E = [Tex]\frac {q}{4 \pi\varepsilon_0}[/Tex]
From the above equation we can say that the electric field outside the shell is similar to the electric field due to a point charge. Thus outside the sphere, the electric field behaves as though it is due to a point charge (carrying all the charge of the shell) at the Centre of the shell.
Electric Field Inside the Shell
If the point P lies inside the spherical shell, then the Gaussian surface is a surface of a sphere having radius r. Since no charge is present inside the spherical shell, the Gaussian surface encloses no charge. Hence, q = 0.
To derive the value you can put the value of q = 0 in the formula E = q/ε0 which will give the result zero.
Electric Field at the Surface of the Shell
At the surface of the shell, r = R
E = q/(4πR2ε0)
If σ is the surface charge density then
q = (4πR2ε0).σ
Since, we have E = q/(4πR2ε0)
Putting value of q = (4πR2ε0).σ we get
E = σ/ε0
Applications of Electric Field
The applications of electric field are mentioned below:
- Electroporation: A technique in which electric fields are used to make pores in cell membranes to insert drugs, medicines, or genes. It is generally used in cloning processes.
- Electric fields play a role in studying tissue dynamics and controlling crystallization processes like nucleation and crystal growth, etc.
- Electric fields are used to impart kinetic energy to charged particles as they travel through the particle accelerator
- Electric fields are used to accelerate charged particles (ions) through a vacuum chamber, allowing scientists to separate ions based on their mass-to-charge ratios
- Electric fields cause polarization of dielectric materials, resulting in the accumulation of electric charge and increased capacitance.
Solved Examples on Electric Field
Example 1. A force of 100 N is acting on the charge 10 μ C at any point. Determine the electric field intensity at that point.
Solution:
Given:
Force F = 100 N
Charge q = 10 μ C
Electric field formula is given by
E = F / q
E = 100N / 10×10−6C
E = 107 N/C.
2. Calculate the electric field at points P, Q for the following two cases.(figure is provided below).
(a) For a charge of +1 µC placed at the origin.
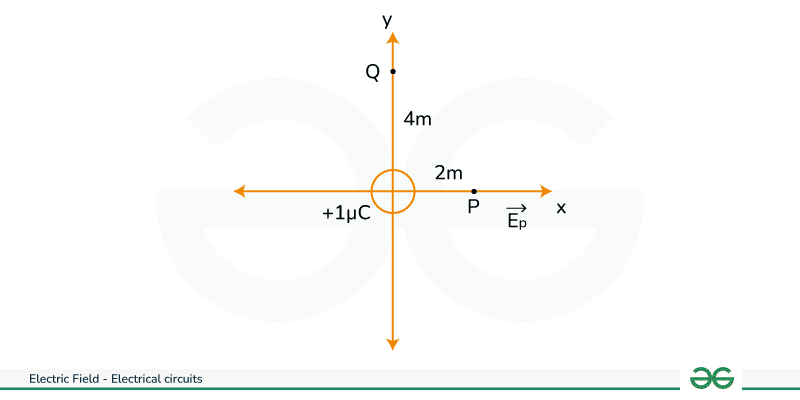
Figure of part a
The magnitude of the electric field at point P is
Ep = {1/4πε }(q/r2 )
Ep = (9 × 109 × 1 × 10-6 )/4 = 2.25 × 103 NC-1
Since, the source charges is positive, the electric field points away from the charge,
So the electric field at the point P is given by
[Tex]\overrightarrow{\rm E}
[/Tex] = 2.25 × 103 NC-1
For the point Q;
EQ = 9 × 109 × 1 × 10-6/16 = 0.56 × 103 NC-1
(b)For a charge of -2 µC placed at the origin
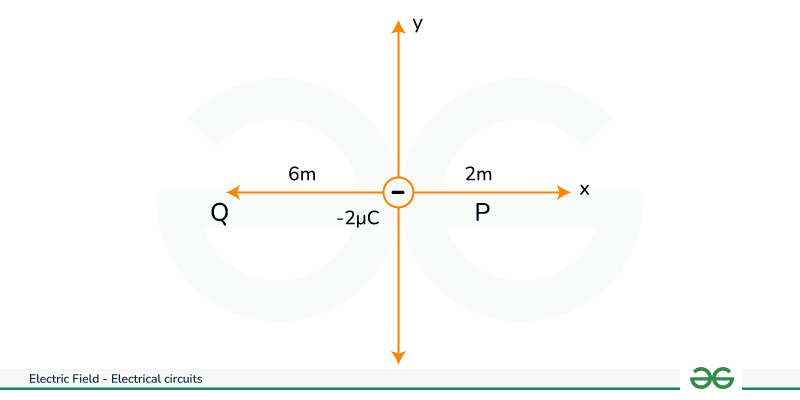
Figure for part -b
The magnitude of the electric field at point P is
Ep = {1/4πε }(q/r2 )
Ep = (9 × 109 × 2 × 10-6)/4
Ep = 4.5 × 103 NC-1
Since, the charge is negative, the electric field points towards,
So, the electric field at point P is given by
[Tex]\overrightarrow{\rm E}
[/Tex] = -4.5 × 103 NC-1
For the point Q = (9 × 109 × 1 × 10-6 )/36 = 0.5 × 103 NC-1
FAQs on Electric Field
What has an electric field?
An electric charge has an electric field
What is an Electric Field?
An electric field at a particular location indicates the force exerted on a unit positive test charge placed at that point.
The electric field formula is given as E = F/q = {1/4πε0}(Q/r2 )
What is electric flux?
Electric flux represents the total number of electric field lines passing through a specified area per unit time.
What is a point charge?
A point charge refers to a theoretical charge situated at a point in space.
What is unit of electric field?
The unit of electric field in NC-1
k is coulomb constant in electric field formula
Share your thoughts in the comments
Please Login to comment...