Chemical Kinetics is a part of physical chemistry that focuses on the rate of chemical reactions and the reasons behind them. It involves figuring out the speed and details of chemical reactions, studying the factors that affect how fast they happen, and how molecules interact during these changes.
This field is important in various scientific areas, from improving industrial processes to understanding complicated reactions in biological systems. By focusing on how molecules interact, chemical kinetics helps us to understand the complex movements of atoms and molecules in the world of chemistry.
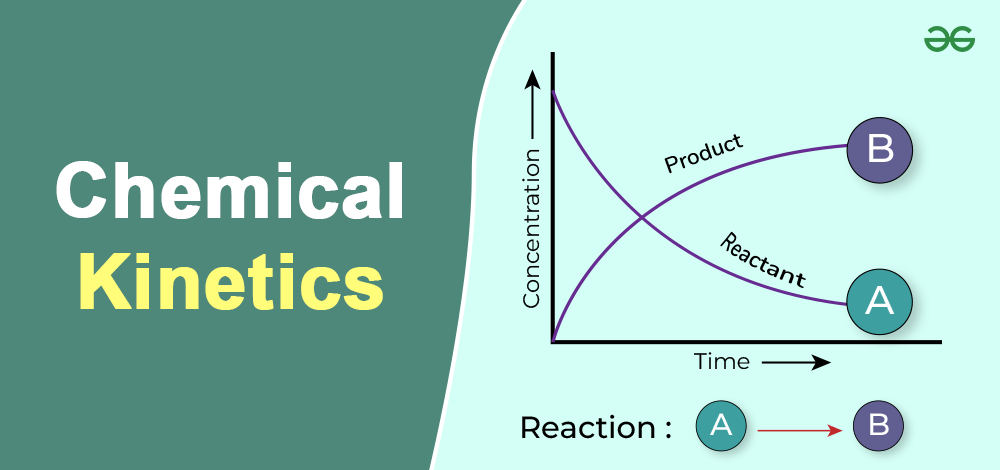
What is Chemical Kinetics?
The term “kinetics” originates from the Greek word ‘kinesis,’ meaning movement. Unlike thermodynamics, which addresses the feasibility of a reaction, chemical kinetics focuses on explaining the rate of a reaction.
The study of chemical kinetics is important because Factors such as concentration, temperature, pressure, and catalysts play pivotal roles in controlling reaction rates. Chemical reactions are often categorized based on their rates.
Examples include fast reactions, such as the reaction of sodium with water (Na + H2O), moderate reactions, like the reaction of magnesium with water (Mg + H2O), and slow reactions, as observed in esterification processes.
What is the Rate of Reaction?
The rate of a reaction, or its speed, is defined as the change in concentration of a reactant or product per unit of time.
Specifically, it can be defined in terms of either the rate of decrease in the concentration of a reactant or the rate of increase in the concentration of a product. In every chemical reaction, as the reaction proceeds, the quantity of reactants decreases while the amount of products rises.
By plotting the concentration of reactants and products against time, one can readily compute the rates of product formation and reactant disappearance based on the slopes of their respective curves. Notably, the overall reaction rate may or may not be equal to the formation and disappearance rates.
Factors Affecting Reaction Rates
The rate of a reaction can be modified by changing certain parameters. Here are the key factors:
Concentration of Reactants: According to collision theory, an increase in the concentration of reactants leads to more collisions between molecules, consequently elevating the reaction rate.
- Example: In a reaction A + B → C, if the concentration of A and B is increased, the rate of the reaction will likely increase due to a higher frequency of collisions.
Nature of the Reactants: The reaction rate is influenced by the types of substances involved. Acid/base reactions and salt formation tend to be fast, while reactions resulting in the formation of larger molecules through covalent bonds are usually slower. The nature and strength of bonds in reactant molecules play a significant role in the rate of transformation into products.
- Example: Acid/base reactions and salt formation often exhibit faster rates. Conversely, the formation of larger molecules through covalent bonds, like in the reaction C6H12O6 → 2C2H5OH + 2CO2, tends to be slower.
Physical State of Reactants: The physical state (solid, liquid, or gas) of a reactant affects the rate of change. Homogeneous phases (e.g., both in an aqueous solution) allow for increased collisions, while different phases limit reactions to interfaces between reactants.
- Example: In the reaction between solid magnesium (Mg) and hydrochloric acid (HCl), the physical state influences the rate. When Mg is in a powdered form, the reaction proceeds faster than when it is in larger solid pieces.
Surface Area of Reactants: Increasing the surface area, such as by crushing a solid into smaller particles, enhances the frequency of collisions between reactant particles, leading to a faster reaction.
- Example: For the reaction 2H2(g) + O2(g) → 2H2O(g), finely divided powdered iron as a catalyst increases the surface area, promoting a faster reaction.
Temperature: An increase in temperature results in more collisions between reactant molecules per second, thereby boosting the reaction rate. Depending on whether the reaction is endothermic or exothermic, temperature influences the rates of forward or backward reactions.
- Example: In the combustion of methane (CH4) with oxygen (O2), increasing the temperature leads to a higher reaction rate, as more collisions occur between the molecules.
Effect of Solvent: The nature of the solvent influences the reaction rate of solute particles. For instance, reactions may occur faster in organic solvents like DMF compared to solvents like methanol due to specific bonding characteristics.
- Example: The reaction of sodium acetate with methyl iodide occurs faster in organic solvents like DMF (dimethylformamide) than in CH3OH (methanol) due to specific bonding characteristics.
Catalyst: Catalysts modify the reaction rate by altering the reaction mechanism. Two types of catalysts, promoters, and poisons, respectively increase and decrease reaction rates.
- Example: The decomposition of hydrogen peroxide (2H2O2) is catalyzed by manganese dioxide (MnO2), increasing the rate of the reaction without being consumed in the process.
Average and Instantaneous Rate
Average Rate of Reaction
The rate of a reaction is categorized into average and instantaneous rates based on the period considered. When the period is finite, it is referred to as the average rate and is denoted as:
ravg = ΔC/Δt
Where ravg average rate, ΔC change in concentration, Δt change in time.
Instantaneous Rate of Reaction
The average rate often needs to provide precise information regarding the completion of a reaction. For instance, consider the hydrolysis of esters into acid and alcohol in the presence of water
RCOOR’ → RCOOH + R’OH
Assuming a 2M solution of ester at time t = 0 which becomes 1M in 30 minutes, one might logically infer that the completion time will be 1 hour. However, in reality, the reaction requires more than 3 hours to complete. To obtain a more comprehensive understanding of the time needed for completion and for other analytical purposes, the “Instantaneous rate” is employed, represented as
rinst = limΔt→0 ΔC/Δt = d[C]/dt
Where rinst is the instantaneous rate.
Unit of Rate of Reaction
The unit of rate is commonly expressed as Molarity per second (Mol s-1) or mol per liter per second (mol/L/s), representing the ratio of concentration to time (mol L-1 divided by seconds).
The units of the rate constant, k, depend on the overall reaction order, and for a zero-order reaction, the units of k are M/s, for a first-order reaction, the units are 1/s, and for a second-order reaction, the units are 1/(M·s)
Rate Law
Rate Law or Law of Mass Action is a principle stating that the rate of a chemical reaction is directly proportional to the product of the masses of the reactants, each raised to a power equal to its coefficient in the balanced chemical equation.
This law was developed by Norwegian scientists Cato M. Guldberg and Peter Waage between 1864 and 1879. This fundamental principle that explains the dynamic equilibrium behavior observed in solutions. Moreover, it also states that the ratio of reactant to product concentrations remains constant at a state of chemical equilibrium.
Equilibrium Constant (Kc)
At equilibrium, the concentrations of reactants and products remain constant at a given temperature. Consider a simple reversible reaction where A and B are reactants, and C and D are products:
aA + bB → cC + dD
An equilibrium mixture, comprising both products and reactants, maintains a specific relationship between their concentrations. This relationship is expressed through the equilibrium constant, Kc, as follows
Kc=[C]c [D]d /[A]a[B]b
Here, Kc represents the equilibrium constant measured in moles per litre.
In this equation, the concentrations of A, B, C, and D at equilibrium are denoted by [A],[B],[C], and[D] respectively, with stoichiometric coefficients for each species.
Order of Reaction
In the rate equation, we know,
Rate = k [A]x [B]y
Where x and y indicate how sensitive the rate is to the change in concentration of A and B.
The sum of these exponents, i.e., x + y gives the overall order of a reaction whereas x and y represent the order concerning the reactants A and B respectively.
Read More about Order of Reaction.
Different types of chemical reactions are categorized based on how the rate of the reaction depends on the concentration of the reactants:
- Zeor Order Reactions
- First-Order Reactions
- Pseudo-First Order Reactions
- Second Order Reactions
Let’s discuss these types in brief as follows:
Zero Order Reactions
The rate of reaction in these reactions is unaffected by the concentration of the reactants. Changes in the concentration of the reactants do not impact the reaction speed.
- Example: Enzyme-catalyzed oxidation of ethanol (CH3CH2OH) to acetaldehyde (CH3CHO).
First-Order Reactions
The rates of these reactions depend on the concentration of only one reactant (order of reaction is 1). Even if multiple reactants are present, only one reactant has a first-order concentration, while the others have zero-order concentration.
- Example: 2H2O2 → 2H2O + O2.
Read more about First Order Reaction.
Pseudo-First Order Reactions
In pseudo-first-order reactions, one reactant’s concentration remains constant and is included in the rate constant in the rate expression. This constant concentration may be due to an excess compared to other reactants or because it acts as a catalyst.
- Example: CH3COOCH3 + H2O → CH3COOH + CH3OH (pseudo-first order kinetics due to excess water).
Read more about Pseudo First Order Reaction.
Second-Order Reaction
A second-order reaction has an order of 2. The rate of these reactions can be expressed as the square of the concentration of one reactant or as the product of the concentrations of two separate reactants.
- Example: r = k[A]2 or r = k[A][B], e.g., NO2+ CO → NO + CO2
Integrated Rate Law
Integrated rate law is a fundamental concept in chemical kinetics that describes the concentration of reactants or products over time during a chemical reaction. It provides a mathematical relationship between the concentration of a reactant or product and time, thereby allowing for the determination of reaction kinetics and rate constants.
Zero-Order Reaction Rate
In a zero-order reaction, the reaction rate is independent of the concentration of the reactant. The integrated rate equation for a zero-order reaction is:
[A]t = −k.t+[A]0
First-Order Reaction Rate
In a first-order reaction, the reaction rate is directly proportional to the concentration of the reactant. The integrated rate equation for a first-order reaction is:
ln[A]t = −k.t +ln[A]0
Second-Order Reaction Rate
In a second-order reaction, the reaction rate is proportional to the square of the concentration of the reactant. The integrated rate equation for a second-order reaction is:
1/[A]t= k.t+1/[A]0
​​Where,
- [A]t is the concentration of the reactant at time t,
- k is the rate constant,
- [A]0 is the initial concentration of the reactant.
Read More about Integrated Rate Laws.
Half-Life of a Reaction
In chemical kinetics, the concept of half-life represents the time required for the concentration of a reactant to decrease to half of its initial value. The half-life is particularly useful in understanding the kinetics of first-order reactions, where the reaction rate is directly proportional to the concentration of the reactant.
For a first-order reaction, the relationship between the half-life (t1/2), the rate constant k, and the initial concentration of the reactant [A0]can be expressed as
t1/2=0.693/k
This equation indicates that the half-life of a first-order reaction is inversely proportional to the rate constant k. As the rate constant increases, the half-life decreases, indicating a faster rate of reaction.
Some Other Terms Related to Chemical Kinetics
Some of the other common related terms to chemical kinetics are:
Let’s discuss these terms in detail as follows.
Activation Energy
In chemical kinetics, activation energy (Ea) defines the energy barrier that must be overcome for a chemical reaction to occur. It represents the minimum amount of energy required for reactant molecules to undergo a successful collision and transform into products. The concept of activation energy is central to the understanding of reaction rates.
Arrhenius Equation
The Arrhenius equation is a mathematical formula that explains the influence of temperature on the pace of a chemical reaction, serving as the basis for predictive models used to determine reaction-rate constants. It is expressed as an exponential function:
k = A. exp(- E/RT)
Where k denotes the reaction-rate constant, A signifies the collision frequency leading to the reaction, E represents the activation energy , R denotes the ideal gas constant (8.314 joules per kelvin per mole), and T is the absolute temperature.
Catalysis
Catalysis is a fundamental concept in chemical kinetics that involves the acceleration of chemical reactions by substances known as catalysts.
Catalysts are substances that facilitate chemical reactions by providing an alternative reaction pathway with a lower activation energy, thereby reducing the energy barrier required for the reaction to occur. There are two main types of catalysis:
- Homogeneous Catalysis: In homogeneous catalysis, the catalyst and the reactants are in the same phase (usually liquid or gas). The catalyst interacts with the reactants to form an intermediate complex, which then proceeds to form the products. The catalyst is typically present in small quantities relative to the reactants.
- Heterogeneous Catalysis: In heterogeneous catalysis, the catalyst is in a different phase from the reactants. For example, the reactants may be in the gas phase while the catalyst is a solid. The reactant molecules adsorb onto the surface of the catalyst, where the reaction takes place. The products then desorb from the catalyst surface.
Catalysis plays a crucial role in various industrial processes, including petroleum refining, chemical synthesis, and environmental remediation.
Collision Theory
Collision Theory is a framework utilized to forecast the rates of chemical reactions, particularly in the context of gases. It states that for a reaction to occur, the reacting entities—be they atoms or molecules—must converge or collide. However, not all collisions result in chemical transformation.
An effective collision leading to chemical change necessitates that the colliding entities possess a minimum level of internal energy, equivalent to the activation energy of the reaction. Additionally, the colliding entities must be arranged in a manner conducive to the required rearrangement of atoms and electrons.
Conclusion: Chemical Kinetics
In conclusion, chemical kinetics stands as a fundamental discipline within the realm of physical chemistry, shedding light on the intricacies of reaction rates and mechanisms. From industrial applications to understanding biological processes, chemical kinetics plays a pivotal role in diverse scientific endeavors. Its significance extends beyond the laboratory, impacting areas such as material synthesis, environmental studies, and pharmaceutical development.
Also Read
Solved Example on Chemical Kinetics
Let’s solve some example problems on Chemical Kinetics
Example 1 : Identify the reaction order from each of the following rate constants.
- k = 2.3 × 10-5 L mol-1 s-1
- k = 3 × 10-4 s-1
Solution:
(1) The unit of second order rate constant is L mol-1 s-1 ,therefore k = 2.3 × 10-5 L mol-1 s-1 represents a second order reaction.
(2) The unit of a first-order rate constant is s-1 therefore k = 3 × 10-4 s-1 represents a first-order reaction.
Example 2: A first-order reaction is found to have a rate constant, k = 5.5 × 10-14 s-1. Find the half-life of the reaction.
Solution:
The half-life for a first-order reaction is t1/2 =0.693/k
So here, t1/2 = 0.693/ (5.5 × 10-14 )s-1 = 1.26 × 1013s
Example 3: Calculate the overall order of a reaction which has the rate expression
- Rate = k [A]1/2 [B]3/2
- Rate = k [A]3/2 [B]-1
Solution:
Rate = k [A]x[B]y
Order = x + y
1. Order = 1/2 + 3/2 = 2, i.e., hence the reaction is of second order
2. Order = 3/2 + (–1) = 1/2, i.e., hence the reaction is of half order.
Chemical Kinetics FAQs
What is Chemical Kinetics?
Chemical kinetics is the branch of physical chemistry focused on understanding the rates of chemical reactions.
Who is the Father of Chemical Kinetics?
Ludwig Ferdinand Wilhelminy, commonly known as Ludwig Wilhelmy, is considered the Father of Chemical Kinetics due to his pioneering work studying the rate of inversion of sucrose in 1850.
Why do we Study Chemical Kinetics?
Chemical kinetics is studied to understand reaction mechanisms, improve control over reactions, optimize industrial processes, construct mathematical models, and contribute to fundamental scientific curiosity.
What is K in Chemical Kinetics?
In chemical kinetics, the symbol K represents the reaction rate constant, which is a proportionality constant that quantifies the rate of a chemical reaction.
What is the Basic Theory of Chemical Kinetics?
The basic theory of chemical kinetics involves understanding the rates of chemical reactions and the factors that influence them.
Share your thoughts in the comments
Please Login to comment...