Benzene is aromatic compound which act as precursor to derive other compounds. Reactions of benzene involve the substitution of a proton by other groups. Electrophilic aromatic substitution is a method of derivatizing benzene. The most common example of this reaction is the ethylation of benzene. Different important reactions of benzene include sulfonation, chlorination, nitration, and hydrogenation. The activating or deactivating effect of substituents on the benzene ring determines the reaction’s direction and the ring’s reactivity.
In this article, we will learn about the different reactions of benzene, along with basic introduction of benzene and its structure.
What is Benzene?
Benzene is a colourless or light yellow, highly flammable liquid with a sweet odor. It consists of six carbon atoms arranged in a planar hexagonal structure, forming a ring with one hydrogen atom attached to each carbon. Benzene is an aromatic hydrocarbon. It possesses unique chemical stability due to the delocalization of its electrons across the ring. This stability makes benzene a versatile building block for various industrial applications, including manufacturing plastics, resins, synthetic fibers, lubricants, dyes, detergents, drugs, and pesticides.
Structure of Benzene
Benzene is a planar molecule of six carbon atoms arranged in a hexagonal ring, with one hydrogen atom attached to each carbon atom. Each carbon-carbon bond in benzene exhibits equal bond lengths, approximately 1.39 angstroms (Å), which is longer than a standard single covalent bond (approximately 1.47 Å) but shorter than a double bond (approximately 1.34 Å).
This unusual bond length arises from the delocalization of electrons over the entire ring, creating a system of alternating single and double bonds, referred to as a resonance hybrid or a delocalized π-electron cloud.
Reactivity of Benzene
The nature of its substituents influences the reactivity of benzene in substitution reactions. Substituents can activate or deactivate the benzene ring, affecting its reactivity in electrophilic aromatic substitution (EAS) reactions. Electron-donating groups, such as -OH and -CH3, activate the benzene ring, increasing its reactivity towards EAS.
In contrast, electron-withdrawing groups, such as -NO2 and -COOH, deactivate the benzene ring, decreasing its reactivity in EAS. The substituents’ nature also influences the substitution position in the benzene ring. Activating groups generally direct substitutions to the ortho and para positions, while deactivating groups direct substitutions to the meta positions.
Reactions of Benzene
Benzene undergoes various reactions, particularly electrophilic aromatic substitution (EAS) reactions. Notably, some of the significant reactions include:
Electrophilic Aromatic Substitution (EAS)
Electrophilic Addition Reactions
- Friedel Craft Alkylation
- Friedel Craft Acylation
Benzene Reduction
Benzene Ring Opening Reaction
- Birch Reduction
- Benzene Oxidation
- Electrophilic Aromatic Substitution
Electrophilic Substitution Reaction
Benzene undergoes Electrophilic Susbtitution Reaction. In these reactions, an electron deficit species that need an electron also called electrophile replaces an atom or group of atoms in benzene to form another compound. Electrophilic Substitution Reaction includes the following reactions let’s discuss them one by one.
Nitration of Benzene
The nitration of benzene is an electrophilic substitution reaction between benzene and a mixture of concentrated nitric acid and concentrated sulfuric acid.
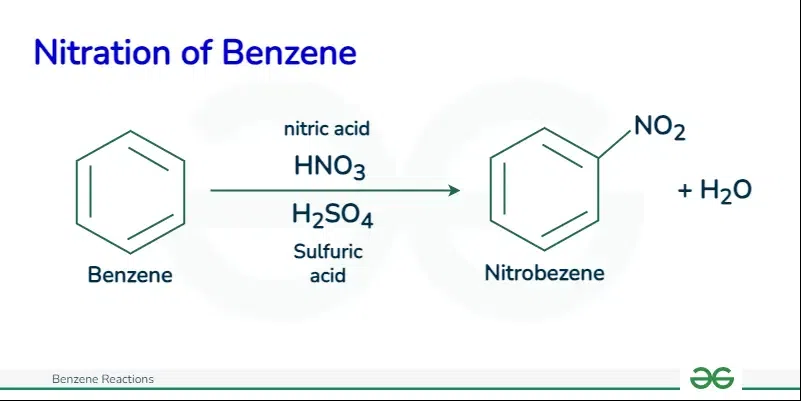
The reaction of nitration of benzene is shown mentioned below:
C6H6 —-Conc. H2SO4 and Conc. HNO3—-> C6H5NO2 + H2O
The mechanism for the nitration of benzene can be summarized as follows:
- Nitric acid takes a proton from sulfuric acid to form the nitronium ion.
- The nitronium ion acts as an electrophile and reacts with benzene to form an arenium ion.
- The arenium ion loses its proton to a Lewis base, forming nitrobenzene.
Sulfonation of Benzene
The sulfonation of benzene proceeds via an electrophilic aromatic substitution mechanism, specifically utilizing sulfur trioxide (SO3) or fuming sulfuric acid (oleum, H2S2O7) containing SO3 as the electrophile.
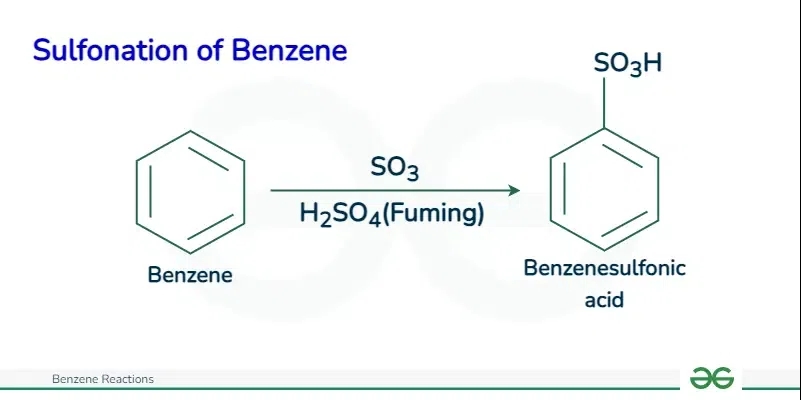
Here is a step-by-step description of the mechanism:
- Generation of the electrophile: In the case of fuming sulfuric acid, SO3 is already present; however, if concentrated sulfuric acid is used, SO₃ is formed through the dissociation of some H2SO4 into SO3 and H₂O.
H2SO4 ⇌ H2O + SO3
- Attack by the electrophile: The electrophilic sulfur atom attracts the electron density within the benzene ring.
- Formation of the sigma complex (arenium ion): An intermediate called the arenium ion is formed when the sulfur atom bonds to a carbon atom in the benzene ring.
- Proton transfer: A proton from the benzene ring moves to the negatively charged oxygen atom of the attached SO3, resulting in the formation of benzenesulfonic acid.
Here is a simplified representation of these steps:
C6H6 + SO3 → C6H5SO3H
Halogenation of Benzene
The halogenation of benzene involves the substitution of a hydrogen atom on the benzene ring with a chlorine or bromine atom. This reaction occurs in the presence of a catalyst such as aluminum chloride or iron. The catalyst helps activate the halogen to become a strong electrophile.
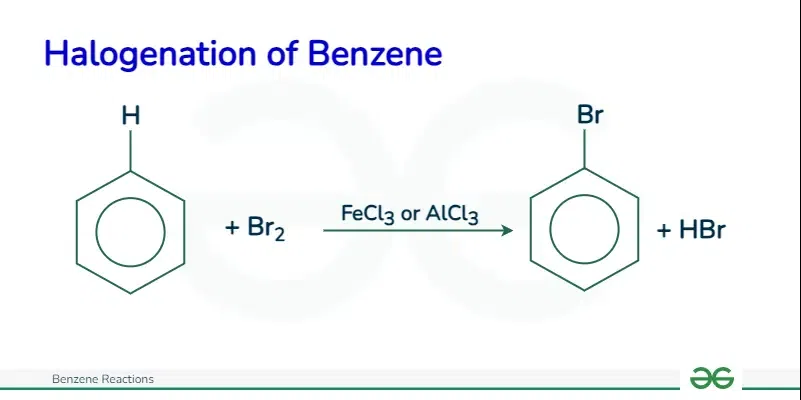
For example, chlorobenzene is formed when benzene reacts with chlorine in the presence of aluminum chloride.
The specific reactions for chlorination and bromination of benzene are as follows:
C6H6 + Cl2 → C6H5Cl + HCl
The mechanism of electrophilic aromatic substitution between benzene and chlorine or bromine involves several steps:
- The halogen molecule approaches the benzene ring, and the delocalized electrons in the ring repel electrons in the halogen-halogen bond.
- The slightly positive end of the halogen molecule acts as the electrophile, facilitated by the presence of the catalyst.
- The electrophilic substitution occurs, leading to the formation of chlorobenzene or bromobenzene.
Electrophilic Addition Reaction
Electrophilic addition reactions involve the interaction between an electrophile and a substance containing a pi bond, forming new sigma bonds. They are crucial in transforming alkenes and alkynes into various functional groups. The process typically occurs in two main steps:
- A carbocation or similar intermediate is formed by the electrophile attacking the pi bond.
- A nucleophilic attack by a suitable nucleophile on the carbocation or related intermediate creates a new sigma bond.
Friedel Craft Alkylation
In this reaction, an alkylating agent, like an alkyl halide, transfers an alkyl group to the aromatic ring in the presence of a strong Lewis acid catalyst, such as aluminum chloride.
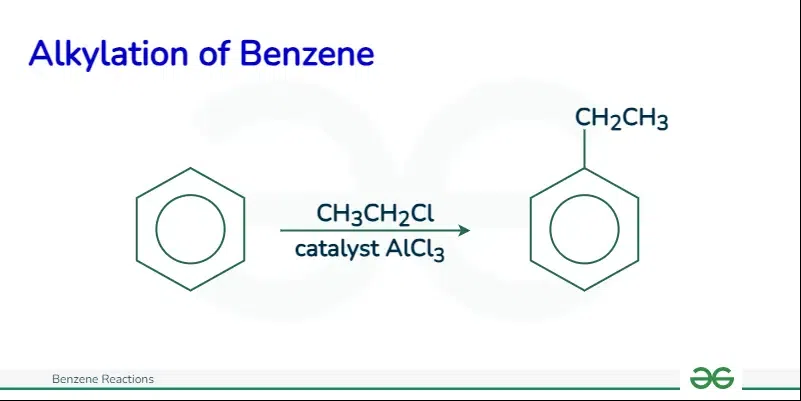
The mechanism of Friedel-Crafts alkylation involves three main steps:
- Generation of the electrophile: The alkyl halide is activated by the Lewis acid catalyst to form a carbocation, which acts as the electrophile.
- Attack of the electrophile: The electrophile is attacked by the π electrons of the aromatic ring, leading to the formation of a new carbon-carbon bond.
- Removal of a proton: A proton is absorbed from the carbon adjacent to the newly added alkyl group, resulting in the formation of the alkylated aromatic product
Friedel Craft Acylation
Friedel-Crafts acylation is a reaction that involves the acylation of aromatic rings using acyl halides and Lewis acids, such as aluminum trichloride, as catalysts.
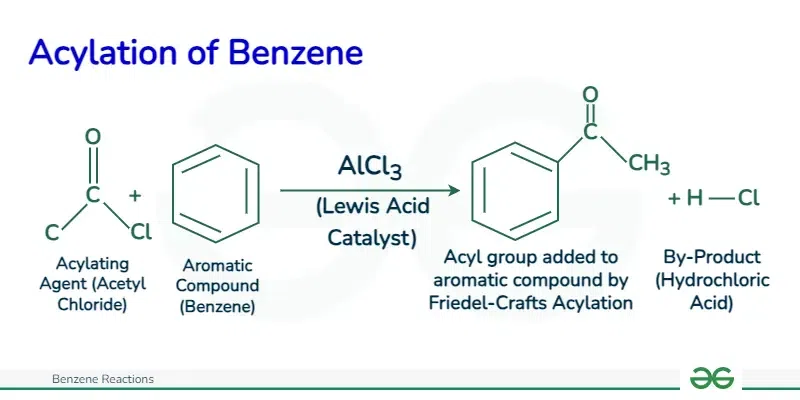
The mechanism of Friedel-Crafts acylation involves three main steps:
- Electrophile Formation: In the first step, the acyl halide (R−C=O−X where X is a halogen such as chloride or bromide) reacts with the Lewis acid catalyst (AlCl3) to form an acylium ion intermediate. This step involves the transfer of a halogen atom from the acyl halide to the Lewis acid, generating an acylium ion (R−C+=O) and a halide ion
- Electrophilic Attack: In the second step, the electrophilic acylium ion (R−C+=O) attacks the benzene ring, leading to the formation of a sigma complex or arenium ion intermediate. This step involves the transfer of the positive charge from the acylium ion to one of the carbon atoms in the benzene ring.
- Removal of Proton: In the final step, the sigma complex undergoes rearrangement to regenerate the aromaticity of the benzene ring. This step involves the loss of a proton (H+) from the sigma complex, resulting in the formation of the substituted benzene product.
Benzene Reduction
Benzene reduction can be achieved through various methods, including catalytic hydrogenation, Birch reduction, and Friedel-Crafts acylation followed by reduction.
Catalytic hydrogenation of benzene requires forcing conditions, such as high heat and hydrogen pressure, to reduce the aromatic ring selectively without affecting other functional groups. Under milder conditions, the double bond of an alkene can be reduced without reducing the aromatic ring.
Benzene Hydrogenation
Benzene hydrogenation is a chemical reaction in which benzene (C6H6) reacts with hydrogen (H2) in the presence of a suitable catalyst to form cyclohexane (C6H12).
The general equation for benzene hydrogenation is:
C6H6 + nH2→C6H12
The reaction proceeds under high pressure and elevated temperature to increase the rate of hydrogenation. This reaction requires a catalyst such as platinum (Pt), palladium (Pd), nickel (Ni), and ruthenium (Ru) to facilitate the reaction.
Benzene Ring Opening Reaction
The ring-opening reaction of benzene is not common or typical, as benzene is known for its stability and resistance to ring-opening. The aromaticity of benzene, resulting from its delocalized pi electrons, makes it highly resistant to ring-opening reactions.
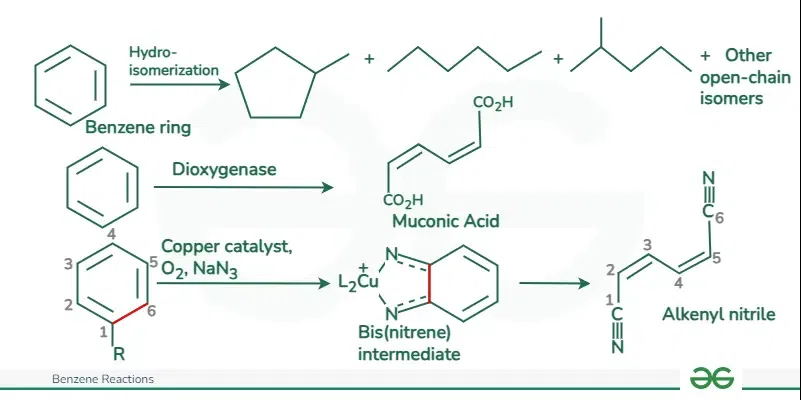
Birch Reduction
The reduction of benzene can be achieved through Birch reduction, which involves using an electron-rich solution of alkali metals, usually lithium or sodium, in liquid ammonia.
The mechanism of the Birch reduction is given below:
- The initial step of the Birch reduction is an electron transfer to the lowest unoccupied molecular orbital of the benzene pi system to form a radical anion.
- Subsequent steps include a sequence of proton- and electron-transfer steps, leading to the reduction of benzene to 1,4-cyclohexadiene
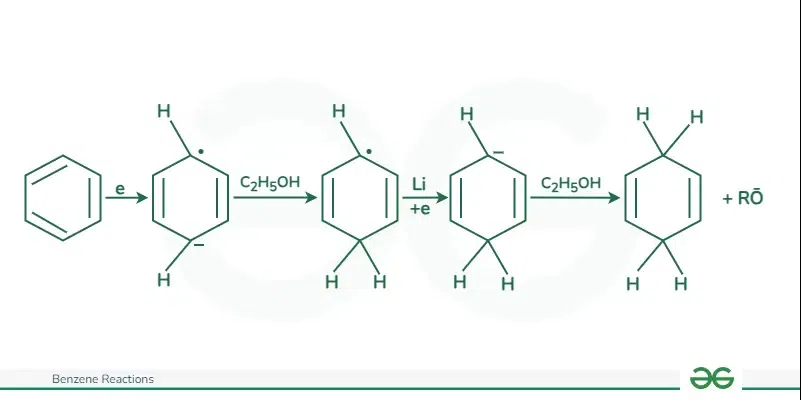
Benzene Oxidation
The oxidation of benzene is a complex process because of the stability and resistance of the aromatic ring to oxidation. However, benzene can be oxidized under extreme conditions or with specific catalysts to form various products.
There are some examples of oxidation of benzene are
- High-Temperature Oxidation: At elevated temperatures, benzene can be partially oxidized to form phenol and other polymeric products like polyphenols and benzoquinones.
- Photocatalytic Oxidation: Using photocatalysts, such as copper-coated palladium nanoparticles, benzene can be highly oxidized to phenol at room temperature.
- Oxidation of Alkylbenzenes: When benzene contains an alkyl group, the alkyl portion is much more prone to oxidation, forming benzoic acids.
- Low-NOx Conditions: Studies have demonstrated that under low-NOx conditions, benzene oxidation produces higher yields of hydroxylated intermediates and lower yields of methane and ethane.
Electrophilic Aromatic Substitution
Electrophilic aromatic substitution (EAS) occurs through a two-step process:
- Formation of a strong electrophile, which attacks the aromatic ring, forming a sigma complex (resonance-stabilized carbocation-like intermediate). This step is rate-determining and involves breaking the aromaticity of the ring.
- Proton transfer from the sigma complex back to the solvent or other base, regenerating the aromatic ring.
This mechanism contrasts with nucleophilic aromatic substitution (NAS), where the aromatic ring acts as an electrophile and forms a negatively charged intermediate before the departure of the leaving group.
Nucleophilic Aromatic Substitution
Nucleophilic aromatic substitution (NAS) is a substitution reaction in organic chemistry in which a nucleophile replaces a leaving group on an aromatic ring. The reaction proceeds through a negatively charged intermediate, and the presence of electron-withdrawing groups on the aromatic ring accelerates the reaction. The reaction is different from a typical SN2 reaction because it occurs at a trigonal carbon atom (sp2 hybridization)
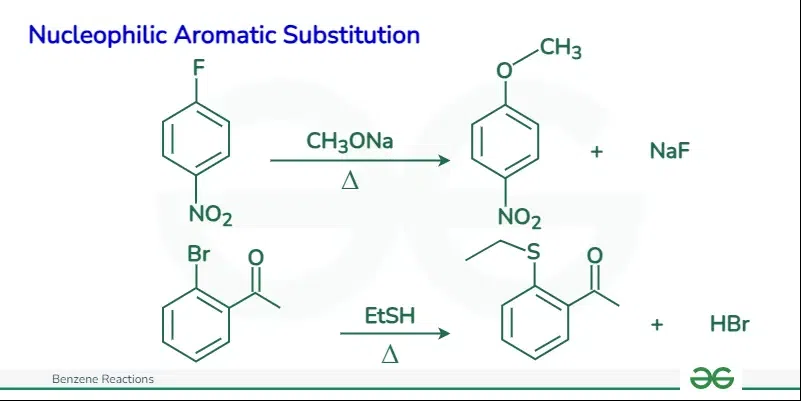
The mechanism steps for nucleophilic aromatic substitution (NAS) involving benzene can be summarized as follows:
- Formation of a negatively charged intermediate (Meisenheimer complex or arenium ion) due to the attack of the nucleophile on the electron-poor aromatic ring. This step is rate-determining and leads to disruption of the aromaticity.
- Departure of the leaving group, restoring the aromatic character of the ring.
More Benzene Reactions
Apart from the above mentioned reactions, there are more some reactions which is essential in chemistry such as Gatterman Koch reaction, mercuration of benzene, ozonolysis of benzene etc.
Gattermann-Koch synthesis
The Gattermann-Koch reaction is a method for producing benzaldehyde from benzene using carbon monoxide (CO) and hydrochloric acid (HCl) in the presence of anhydrous aluminum chloride (AlCl₃)
Step 1: Formation of formyl chloride
CO HCl ⇌ HCOCl
Step 2: Formation of electrophile (H-C≡O)
HCOCl AlCl3 ⇌ (H C≡O)+Cl− + AlCl2−
Step 3: Formation of benzaldehyde
(H C≡O)+ + Benzene → Benzaldehyde + HCl
Gattermann Aldehyde Reaction
Gattermann aldehyde synthesis is an organic reaction used to synthesize aromatic aldehydes. The reaction involves the conversion of aromatic compounds into aldehydes using hydrogen cyanide, hydrogen chloride, and a Lewis acid such as aluminum chloride.
Step 1. Formation of Formimino Chloride
HCN + HCl⟶ Formimino Chloride
Step 2. Formation of Electrophile
Formimino Chloride + Lewis Acid ⟶ Formimino Cation
Step 3. Attack of Electrophile on Benzene Ring
Formimino Cation + Benzene ⟶ Benzylamine
Step 4. Hydrolysis of Benzylamine
Benzylamine ⟶ Benzaldehyde + Ammonia
Mercuration of Benzene
The mercuration of benzene involves adding a mercury-containing group to the benzene ring. This reaction is typically carried out using mercuric acetate or mercuric chloride in the presence of a Lewis acid catalyst such as aluminum chloride.
The general reaction for the mercuration of benzene :
Benzene + Hg (II) X2 → Mercury Benzene Complex
Blanc Chloromethyation
The Blanc chloromethylation is also called the Blanc reaction. It is a chemical process that introduces a chloromethyl group on aromatic rings. This reaction involves treating aromatic compounds with formaldehyde in the presence of a Lewis acid catalyst such as zinc chloride.
The general reaction for blanc-chloromethylation
Aromatic compound + Formaldehyde + ZnCl2 → Chloromethylated product
Combustion Reaction of Benzene
The combustion reaction of benzene involves the reaction of benzene (C₆H₆) with oxygen (O₂) to produce carbon dioxide (CO₂) and water (H₂O). The balanced chemical equation for the combustion of benzene is as follows:
C6H6 + 15 1/2 O2 → 6CO2 + 3H2O
This balanced equation indicates that one mole of benzene reacts with 15.5 moles of oxygen to produce 6 moles of carbon dioxide and 3 moles of water.
Ozonolysis of Benzene
The ozonolysis of benzene involves reacting with ozone under specific conditions to form organic compounds.
The conditions for the ozonolysis of benzene are as follows:
- Benzene is treated with ozone in the presence of an inert solvent like chloroform (CHCl₃) or carbon tetrachloride (CCl₄) at low temperatures ranging from 196 to 200 Kelvin.
- The reaction forms benzene triozonide as an intermediate, which yields three moles of glyoxal upon reduction with zinc dust and water.
Ozonolysis is a reaction where an alkene or alkyne reacts with ozone to form organic compounds by replacing the multiple carbon-carbon bonds with a double bond to oxygen. In the case of benzene, ozonolysis leads to the formation of glyoxal through the production of benzene triozonide as an intermediate.
Benzene with Metal Complex
Metal complexes of benzene have been extensively studied in organometallic chemistry. These complexes are derivatives of benzene, where a transition metal atom replaces a CH center. The metal complexes of benzene can be classified into two categories:
- Complexes with transition metals
- Complexes with main-group metals
Disubstituted Benzene Reactions
Disubstituted Reactions of benzene refer to reactions where two substituents are introduced onto the benzene ring. These reactions can occur through various mechanisms, including electrophilic aromatic substitution, nucleophilic aromatic substitution, or radical substitution.
The general mechanism for a disubstituted reaction of benzene via electrophilic aromatic substitution involves two sequential electrophilic substitution reactions.
- First Substitution: Benzene undergoes the first electrophilic aromatic substitution reaction to introduce the first substituent. This step involves the generation of an electrophile (E+) and the formation of an arenium ion intermediate. The electrophile attacks the benzene ring, leading to the formation of a sigma complex.
- Second Substitution: The intermediate formed in the first step now undergoes a second electrophilic aromatic substitution reaction to introduce the second substituent. This step involves the generation of another electrophile and the formation of a second arenium ion intermediate. The electrophile attacks the benzene ring at an ortho, meta, or para position relative to the first substituent, thus forming a disubstituted benzene product
Benzylic Position and Impact on Benzene Reactivity
The benzylic position refers to the carbon adjacent to an aromatic ring, particularly in benzene. This position is characterized by increased reactivity due to the resonance stabilization of the benzylic carbocation, which results from the delocalization of the positive charge onto the aromatic ring.
The benzylic position plays a significant role in many types of reactions such as:
- Free radical bromination of alkyl benzenes
- Oxidation of alkyl benzenes
- Substitution reactions of benzylic halides
- Reduction of the benzylic carbon
- Nucleophilic aromatic substitutions
- Electrophilic aromatic substitutions
These reactions are influenced by factors such as the electronic nature of the substituents attached to the aromatic ring, which can affect the overall reactivity of the benzylic position.
Uses of Benzene Reactions
Benzene Reactions are important in chemistry for formation of numerous compounds. Here are few uses of benzene reactions:
- Production of Polymers: Benzene serves as a precursor in the production of polymers such as polystyrene, styrene-butadiene rubber (SBR), and polyethylene terephthalate (PET).
- Synthesis of Pharmaceuticals: Benzene derivatives are crucial components in the synthesis of pharmaceuticals. Many drugs, including painkillers, antihistamines, antibiotics, and anticancer agents, contain benzene-derived structures in their chemical compositions.
- Manufacture of Solvents: Benzene is used as a solvent in various industries, including pharmaceuticals, chemicals, paints, and coatings. Its excellent solvent properties make it valuable for dissolving and dispersing substances.
- Fuel Additive: Benzene is added to gasoline to increase octane levels and improve engine performance.
- Production of Dyes: Benzene-derived compounds are essential in the synthesis of dyes and pigments used in textiles, printing, paints, and cosmetics.
- Aromatic Chemicals: Benzene derivatives serve as building blocks in the synthesis of aromatic chemicals used in fragrances, flavourings, and personal care products.
- Laboratory Reagents: Benzene and its derivatives are commonly used as reagents in organic synthesis in laboratory settings for various research and experimental purposes.
Benzene Reactions FAQs
What is the molecular formula of benzene?
The general molecular formula for benzene is C₆H₆.
What are the three types of substitution reactions of benzene?
The three main types of substitution reactions of benzene are electrophilic aromatic substitution, nucleophilic aromatic substitution, and free radical substitution.
What are the nucleophilic aromatic reactions?
Nucleophilic aromatic substitution is a type of reaction where a nucleophile replaces a leaving group on a benzene ring.
Why is the reaction of benzene essential in organic chemistry?
Reactions of benzene are essential in organic chemistry because benzene serves as a fundamental building block in many organic compounds.
What are the differences between electrophilic substitution and electrophilic addition reactions?
Electrophilic substitution reactions involve the replacement of an atom or group in a molecule by an electrophile. This commonly occurs in aromatic compounds like benzene.
On the other hand, electrophilic addition reactions involve the addition of an electrophile and a nucleophile to a molecule. This is typical in unsaturated compounds like alkenes or alkynes.
What are the differences between electrophilic substitution and nucleophilic substitution reactions?
Electrophilic substitution involves the replacement of an atom or group in a molecule by an electrophile, commonly observed in aromatic compounds.
On the other hand, nucleophilic substitution occurs when a nucleophile replaces a leaving group, typically observed in aliphatic compounds.
What is birch reduction?
Birch reduction is a chemical reaction that involves the reduction of aromatic rings, typically benzene, using liquid ammonia (NH₃) and alkali metals like sodium (Na).
What are the applications of benzene reaction?
Benzene reactions have widespread applications in organic synthesis, producing various chemicals, pharmaceuticals, and materials. Nitration of benzene is used to make explosives like TNT. Sulfonation is employed in the synthesis of detergents and dyes. Friedel-Crafts reactions contribute to the synthesis of fragrances and pharmaceuticals.
Share your thoughts in the comments
Please Login to comment...